Photosynthesis- Process & Reaction
Introduction
Photosynthesis is a process by which most plants, algae, and cyanobacteria (called photoautotrophs) convert light energy into chemical energy. Through cellular respiration, this energy can later be released to fuel the growth and maintenance activities of organisms.
Some of this chemical energy is stored in carbohydrate molecules, such as sugars and starches, which are synthesized from carbon dioxide and water, hence the name photosynthesis, from the Greek phōs “light”, and synthesis, “putting together”.
Photosynthesis is greatly responsible for producing and maintaining the oxygen content of the Earth’s atmosphere, and supplies most of the energy necessary for life on Earth.
The process of photosynthesis can be summarized into following points:
- The energy from light is absorbed by proteins (called reaction centers) held inside chloroplasts, which are most abundant in leaf cells, while in bacteria they are embedded in the plasma membrane.
- In these light-dependent reactions, some energy is used to strip electrons from water, producing oxygen gas. Whereas, the hydrogen freed by the splitting of water is used in the creation of two compounds named nicotinamide adenine dinucleotide phosphate (NADPH) and adenosine triphosphate (ATP), the “energy currency” of cells. These compounds further drive other reactions.
- In plants, algae and cyanobacteria, sugars are synthesized by light-independent reactions called the Calvin cycle. In this cycle, atmospheric carbon dioxide is incorporated into organic carbon compounds, such as ribulose bisphosphate (RuBP). Using the ATP and NADPH produced by the light-dependent reactions, the resulting compounds are then reduced and removed to form carbohydrates, such as glucose.
Photosynthesis and Cellular Respiration
- Photosynthesis is a process of reduction of carbon dioxide to carbohydrates; whereas, cellular respiration is the oxidation of carbohydrates to carbon dioxide.
- Nutrients used in cellular respiration include carbohydrates, amino acids and fatty acids. These nutrients are oxidized to produce carbon dioxide and water, and to release chemical energy to drive the organism’s metabolism.
- Photosynthesis and cellular respiration are distinct processes, as they take place through different sequences of chemical reactions and in different cell organelles.
- The general equationfor photosynthesis was first proposed by Cornelis van Niel is:
CO2 + 2H2A (electron donor) + photons (light energy) → [CH2O] Carbohydrate + 2A (oxidized electron donor) + H2O (water)
Since water is used as the electron donor in oxygenic photosynthesis, the equation for this process is:
CO2 (carbon dioxide) + 2H2O (water) + photons → [CH2O] carbohydrate + O2 (oxygen) + H2O (water)
Here water is both a reactant in the light-dependent reaction and a product of the light-independent reaction, but canceling n water molecules from each side gives the net equation:
CO2 (carbon dioxide) + H2O (water) + photons (light energy) → [CH2O] (carbohydrate) + O2 (oxygen)
Stages of Photosynthesis
- Light-dependent reactions or light reactions: capture the energy of light and use it to make the hydrogen carrier NADPH and the energy-storage molecule ATP. Most organisms that use oxygenic photosynthesis, use visible light for the light-dependent reactions.
- Light-independent reactions or Dark reaction: During the second stage, products of 1st stage are used to capture and reduce carbon dioxide.
Photosynthetic Membranes & Organelles
- In plants and algae, photosynthesis takes place in chloroplasts. A typical plant cell contains about 10 to 100 chloroplasts. The chloroplast is enclosed by a membrane composed of phospholipid inner membrane, phospholipid outer membrane, and an intermembrane space. Enclosed by the membrane is an aqueous fluid called the stroma. Embedded within the stroma are stacks of thylakoids (grana), which are the site of photosynthesis. The thylakoid itself is enclosed by the thylakoid membrane, and within the enclosed volume is a thylakoid lumen. photosynthetic system is found here.
- Plants absorb light primarily using the pigment chlorophyll. The green part of the light spectrum is not absorbed but is reflected which is the reason that most plants have a green color. Besides chlorophyll, plants also use pigments such as carotenes and xanthophylls.
- Algae also use chlorophyll, but various other pigments are present, such as phycocyanin, carotenes, and xanthophylls in green algae, phycoerythrin in red algae and fucoxanthin in brown algae and diatoms resulting in a wide variety of colors. These pigments are embedded in plants and algae in complexes called antenna molecule or light-harvesting complex (LHC).
- Although all cells in the green parts of a plant have chloroplasts, the majority of those are found in leaves. Certain species like Euphorbia and cactus species adapted to conditions of strong sunlight and aridity, have their main photosynthetic organs in their stems. The cells in the interior tissues of a leaf called the mesophyll, can contain between 450,000 and 800,000 chloroplasts for every square millimeter of leaf.
- Leaf surface has waxy cuticle that protects the leaf from excessive evaporation of water and decreases the absorption of ultraviolet or blue light to minimize heating. The epidermis layer allows light to pass through to the palisade mesophyll cells where most of the photosynthesis takes place.
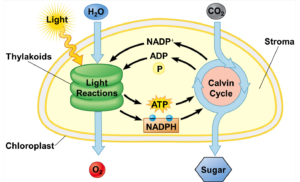
Light Dependent Reaction
- In the light-dependent reactions, one molecule of the pigment chlorophyll absorbs one photon and loses one electron. This electron is taken up by pheophytin, which passes the electron to a quinone molecule, starting the flow of electrons through electron transport chain that leads to the ultimate reduction of NADP to NADPH.
- This creates a proton gradient (energy gradient) across the chloroplast membrane, which is used by ATP synthase in the synthesis of ATP. The chlorophyll molecule ultimately regains the electron it lost when a water molecule is split in a process called photolysis, which releases oxygen.
- The overall equation for the light-dependent reactions under the conditions of non-cyclic electron flow in green plants is:
2 H2O + 2 NADP+ + 3 ADP + 3 Pi + light → 2 NADPH + 2 H+ + 3 ATP + O2
- The photosynthetic action spectrum depends on the type of accessory pigments present and, not all wavelengths of light can support photosynthesis. In green plants, the action spectrum resembles the absorption spectrum for chlorophylls and carotenoids with absorption peaks in violet-blue and red light.
- VIBGYOR: 390-760nm, PAR (Photosynthetically Active Radiation): 400-700nm.
- In red algae, the action spectrum is blue-green light, which allows these algae to use the blue end of the spectrum to grow in the deeper waters that filter out the longer wavelengths (red light) used by above-ground green plants.
- The non-absorbed part of the light spectrum is what gives photosynthetic organisms their color (e.g., green plants, red algae, purple bacteria) and is the least effective for photosynthesis in the respective organisms.
What is Z SCHEME?
The light-dependent reactions are of two forms: cyclic and non-cyclic.
In the non-cyclic reaction, the photons are captured in the light-harvesting complex of “photosystem II” by chlorophyll and other accessory pigments. The absorption of a photon by the antenna complex loosens an electron that is taken up by the primary electron-acceptor molecule (pheophytin). As the electrons are shuttled through an electron transport chain (the so-called Z-scheme shown in the diagram), a chemiosmotic potential is generated by pumping proton cations (H+) across the membrane and into the thylakoid space.
An ATP synthase enzyme uses that chemiosmotic potential to make ATP during photophosphorylation, whereas NADPH is a product of the terminal redox reaction in the Z-scheme. The electron enters a chlorophyll molecule in “Photosystem I”. There it is further excited by the light absorbed by that photosystem. The electron is then passed along a chain of electron acceptors to which it transfers some of its energy. The energy delivered to the electron acceptors is used to move hydrogen ions across the thylakoid membrane into the lumen.
The electron is eventually used to reduce the co-enzyme NADP with an H+ to NADPH (which has functions in the light-independent reaction); at that point, the path of that electron ends.
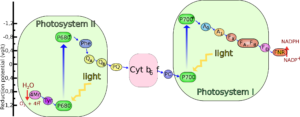
The cyclic reaction generates only ATP, and no reduced NADP (NADPH) is created. The cyclic reaction takes place only at “photosystem I”. Once the electron is displaced from the photosystem, the electron is passed down the electron acceptor molecules and returns to “photosystem I”, from where it was emitted, hence the name cyclic reaction.
Photolysis of water
- “Photosystem II”, requires an external source of electrons to reduce its oxidized chlorophyll a (Reaction center).
- The source of electrons for photosynthesis in green plants and cyanobacteria is water.
- Two water molecules are oxidized by the “photosystem II” to yield a molecule of diatomic oxygenand four hydrogen The electrons yielded are transferred to a redox-active tyrosine residue that is oxidized by the energy of P680+. This resets the ability of P680 to absorb another photon and release another photo-dissociated electron.
- The oxidation of water is catalyzed in “photosystem II” by a redox-active structure that contains four manganese ions and a calcium ion; this oxygen-evolving complex binds two water molecules and contains the four oxidizing equivalents that are used to drive the water-oxidizing reaction.
- The hydrogen ions are released in the thylakoid lumen and therefore contribute to the transmembrane chemiosmotic potential that leads to ATP synthesis.
- Oxygen is a waste product of light-dependent reactions. However, majority of organisms on Earth use oxygen and its energy for cellular respiration.
Light Independent Reaction
Calvin cycle
- In the light-independentor “dark reactions”, the enzyme RuBisCO captures CO2 from the atmosphere and uses NADPH and releases three-carbon sugars, which are later combined to form sucrose and starch.
The overall equation for the light-independent reactions in green plants is:
3 CO2 + 9 ATP + 6 NADPH + 6 H+ → C3H6O3-phosphate + 9 ADP + 8 Pi + 6 NADP+ + 3 H2O
- Carbon fixation produces the 3-carbon sugar intermediate, which is then converted into the final carbohydrate products. The simple carbon sugars produced by photosynthesis are then used to form other organic compounds, like cellulose, the precursors for lipid and amino acid biosynthesis, or as a fuel in cellular respiration.
- The reduction of CO2 is a process in which carbon dioxide combines with a 5-carbon sugar, ribulose 1, 5-bisphosphate, to yield two molecules of a 3-carbon compound (3-phosphoglycerate or Glycerate 3-phosphate). In the presence of ATP and NADPH produced during the light-dependent stages, Glycerate 3-phosphate is reduced to triose phosphate called as glyceraldehyde 3-phosphate or 3-phosphoglyceraldehyde (PGAL). 5 out of 6 molecules of the glyceraldehyde 3-phosphate produced, are used to regenerate ribulose 1, 5-bisphosphate so the process can continue.
Carbon fixation (C4, C3 & CAM plants)
- In hot and dry conditions, plants close their stomata to prevent water loss. Under these conditions, CO2will decrease and oxygen gas, produced by the light reactions will increase, causing an increase of photorespiration by the oxygenase activity of ribulose-1,5-bisphosphate carboxylase/oxygenase and decrease in carbon fixation. Some plants have evolved mechanisms to increase the CO2 concentration in the leaves under these conditions.
- Plants that use the C4 carbon fixation process chemically fix CO2 in the mesophyll cell by adding it to the 3-carbon molecule phosphoenolpyruvate (PEP) catalyzed by an enzyme called PEP carboxylase, creating the 4-carbon organic acid oxaloacetic acid. These organic acids then translocated to bundle sheath cells where the enzyme RuBisCO and other Calvin cycle enzymes are located, and where CO2 released by decarboxylation of the 4-carbon acids is then fixed by RuBisCO activity to the 3-carbon 3-phosphoglyceric acids. The physical separation of RuBisCO from the oxygen-generating light reactions reduces photorespiration and increases CO2 fixation and, thus the photosynthetic capacity of the leaf.
- C4 plants can produce more sugar than C3 plants in conditions of high light and temperature. Examples of C4 plants are maize, sorghum, sugarcane, and millet.
- C3 plants do not use PEP-carboxylase in carbon fixation because the primary carboxylation reaction, catalyzed by RuBisCO, produces the 3-carbon 3-phosphoglyceric acids directly in the Calvin-Benson cycle. Examples of C3 plants are Rice, wheat, barley and soyabean
- Over 90% of plants use C3 carbon fixation, compared to 3% that use C4 carbon fixation.
- Crassulacean acid metabolism (CAM): Xerophytes, such as cacti and most succulents, also use PEP carboxylase to capture CO2 in a process called Crassulacean acid metabolism (CAM). CAM plants have a different leaf anatomy from C3 plants, and fix the CO2 at night when their stomata are open.
- CAM plants store the CO2mostly in the form of malic acid via carboxylation of phosphoenolpyruvate to oxaloacetate, which is then reduced to malate. Decarboxylation of malate during the day releases CO2 inside the leaves, thus allowing carbon fixation to 3-phosphoglycerate by RuBisCO.
- CAM is used by 16,000 species of plants.
See…Difference between C3, C4 & CAM Plants
Photosynthetic Efficiency
Plants usually convert light into chemical energy with a photosynthetic efficiency of 3–6% only. Absorbed light that is unconverted is dissipated primarily as heat, with a small fraction (1–2%) re-emitted as chlorophyll fluorescence at longer (redder) wavelengths.
Photosynthetic efficiency varies with the frequency of the light being converted, light intensity, temperature and proportion of CO2 in the atmosphere, and can vary from 0.1% to 8%.
Factors Affecting Photosynthesis
Light intensity and wavelength
- Light is one of the major factors as the process of photosynthesis cannot occur in the dark and the source of light for the plants is sunlight.
- Photosynthesis begins at low intensities of light and increases till it reaches brightest time of the day. However, the amount of light required varies for different plants.
- Photosynthesis uses maximum up to 5 % light in the process and so light is generally not a limiting factor at high intensity. However, the light becomes a limiting factor in low intensity irrespective of water or CO2 content, as without light photosynthesis cannot occur.
- At high intensities, the temperature of the plant increases and thus increased transpiration in the plant. This leads to the closing of the stomata resulting in reduced CO2 Excessive light inhibits photosynthesis.
- Chlorophyll most effectively absorbs red and blue wavelengths from the entire spectrum of light. Hence, maximum photosynthesis occurs when the plant is exposed to the light of these wavelengths. The longer the plant is exposed to light, the longer the process of photosynthesis will continue.
Carbon dioxide concentration
Plants take CO2 from the atmosphere. The atmosphere contains 0.03% of carbon dioxide, acts as a limiting factor for photosynthesis. When light and temperature are not the limiting factors, increasing CO2 concentration leads to an increase in the rate of photosynthesis. But, beyond a certain limit, CO2 starts accumulating in the plant and this leads to slowing down of the process.
Temperature
Experiments shows that, when CO2 and light are not limiting factors, the rate of photosynthesis increases with increase in temperatures till the optimum level for that plant. Beyond the optimum levels, the enzymes are deactivated and photosynthesis stops.
Water
When there is a reduced water intake or availability, the stomata begin to close to avoid loss of any water during transpiration. With the stomata closing down the CO2 intake also stops which affects photosynthesis.
Oxygen
Oxygen is needed for photorespiration in C3 plants. The energy generated during the oxygen respiration is needed for the process of photosynthesis. However, an increase in the oxygen levels beyond the optimum for the plant leads to inhibition of photosynthesis. This is because oxygen tends to break down the intermediaries that are formed in photosynthesis. Oxygen also completes with CO2 to combine with RUBISCO (dark reaction) of photosynthesis and photorespiration. An increased levels of O2 would mean that RUBISCO will combine with O2 to initiate photorespiration and photosynthesis will slow down.
Discovery & Experiment
- Jan van Helmont (mid-17th century) measured the mass of the soil used by a plant and the mass of the plant as it grew. After noticing that the soil mass changed very little, he hypothesized that the mass of the growing plant must come from the water, the only substance he added to the potted plant. However, his hypothesis was partially accurate as much of the gained mass comes from CO2 as well as water.
- Joseph Priestley (1770) discovered that green plants restore air quality. He isolated a volume of air under an inverted jar and burned a candle in it (which gave off CO2). He discovered that a mouse could injure air. He showed that the air that had been injured by the candle and the mouse could be restored by a plant.
- Jan Ingenhousz(1779) repeated Priestley’s experiments. He discovered that it was the influence of sunlight on the plant that could cause it to revive a mouse in a matter of hours. Plants receive some energy from light in addition to air, soil, and water was first discovered by him.
- Jean Senebier (1796), demonstrated that green plants consume carbon dioxide and release oxygen under the influence of light.
- Nicolas-Théodore de Saussure showed that the increase in mass of the plant as it grows is not only due to uptake of CO2 but also due to the incorporation of water. Thus, the basic reaction by which photosynthesis is used to produce food (such as glucose) was outlined.
- Cornelis Van Niel, by studying purple sulfur bacteria and green bacteria he was the first to demonstrate that photosynthesis is a light-dependent redox reaction, in which hydrogen reduces (donates its atoms as electrons and protons to) carbon dioxide.
- Robert Emerson discovered two light reactions by testing plant productivity using different wavelengths of light. With the red alone, the light reactions were suppressed. When blue and red were combined, the output was much more substantial. Thus, there were two photosystems, one absorbing up to 600 nm wavelengths (PSII), the other up to 700 nm (PSI). PSI contains only chlorophyll “a”, PSII contains primarily chlorophyll “a” with most of the available chlorophyll “b”, among other pigments.
- Robert Hill (1937 & 1939), performed experiments to prove that the oxygen developed during the photosynthesis of green plants came from water. He showed that isolated chloroplasts give off oxygen in the presence of unnatural reducing agents like iron oxalate, ferricyanide or benzoquinone after exposure to light. In the Hill reaction:
2 H2O + 2 A + (light, chloroplasts) → 2 AH2 + O2
A is the electron acceptor. Therefore, in light, the electron acceptor is reduced and oxygen is evolved.
- Samuel Ruben and Martin Kamen used radioactive isotopes to determine that the oxygen liberated in photosynthesis came from the water.
- Melvin Calvin and Andrew Benson, along with James Bassham, explained the path of carbon assimilation in plants. The carbon reduction cycle is known as the Calvin cycle, also refer to it as the Calvin-Benson, Benson-Calvin Cycle.
- Rudolph A. Marcus (Nobel laureate) discover the function and significance of the electron transport chain.
- Otto Heinrich Warburg and Dean Burk discovered the I-quantum photosynthesis reaction that splits CO2, activated by the respiration.
- Otto Kandler (1950) presented first experimental evidence for the existence of photophosphorylation in vivo. In 1954, Daniel I. Arnon et al. discovered photophosphorylation in vitro in isolated chloroplasts with the help of P32.